Monitoring Plant Cell Cultures with BioLector and Multisizer 4e Instruments
Introduction
Cell cultures can be used to grow plant cells. Typically, this technique is performed in an aseptic and controlled environment with a variety of nutrient solutions.1 Plant cell cultures have a wide range of applications from basic research (e.g., studies on plant growth and differentiation) to mass production of plant-derived metabolites (e.g. production of the anti-cancer agent paclitaxel).2 Therefore, they have become increasingly attractive and cost-effective alternatives to classical approaches for the mass production of plant-derived metabolites.3 However, it is crucial to monitor culture conditions to optimize the rate of cell proliferation and maximize productivity levels. Likewise, the cells must be characterized to detect changes in the cell culture, such as the formation of cell aggregates or cellular lysis. This application note will demonstrate how the BioLector microbioreactor and the Multisizer 4e Coulter Counter can be used to optimize plant cell culture conditions and therefore cell growth (Figure 1).
FIGURE 1: The BioLector microbioreactor and the Multisizer 4e Coulter Counter can be used to monitor plant cell cultures.
Monitoring Optimal Cell Culture Conditions
The BioLector microbioreactor is a small-scale, automated system that enables high-throughput screening, cultivation parameter monitoring (e.g., pH, biomass, oxygen saturation, shaking speed, and fluorescence intensity) and feeding strategy optimization. Importantly, all these parameters are monitored online without the necessity to stop shaking or to take samples. In this example, the BioLector device was used to evaluate the influence of different carbon sources and inoculation volumes on the final biomass concentration of plants.
Methods
Cultivations of Nicotiana tabacum BY-2 transgenic cells expressing green fluorescent protein (GFP) were carried out in a 48 well microtiter plate (part no. M2P-MTP-R48-BOH1) of the BioLector instrument. Preculturing was performed by inoculating 180 mL fresh media (pH = 5.8) and 100 mg kanamycin sulfate with 20 mL of a seven-day-old culture in a 250 mL shake flask at 190 rpm (d0 = 50 mm) at 20 °C. The culture medium was composed of 4.4 g/L MSMO (Murashige and Skoog basal salts with minimal organics, Sigma 6899), 0.2 g/L potassium dihydrogen orthophosphate, 0.2 mg/L 2,4-dichlorphenoxyacetic acid, 0.6 mg/L thiamine and 30 g/L carbon sources. Main culturing was carried out in the BioLector microbioreactor at 1000 rpm shaking frequency (d0 = 3 mm) and 26 °C. Experiment 1 tested the proof of concept of the BioLector device. For this, five single wells were inoculated with 10 % (v/v) preculture and scattered light (biomass concentration), two fluorescence signals (GFP and NAD(P)H), pH and DO (dissolved oxygen) were measured. In experiment 2, the effect of different carbon source and inoculation volumes were investigated. Hence, glucose, fructose or saccharose were utilized in the same concentrations (30 g/L carbon source) and the wells were inoculated with either 5 or 10 % (v/v) of preculture.
Results and Discussions
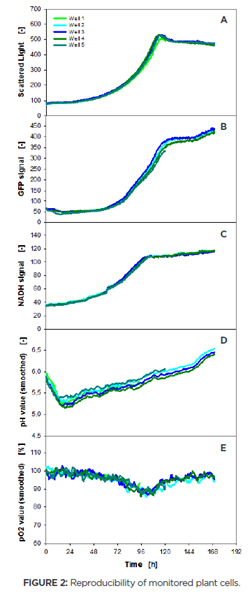
Experiment 2 aimed to evaluate the effect of different carbon sources and inoculation volumes. The results are shown in Figure 3. The biomass concentration (measured as scattered light and/or NAD(P)H signal) at the end of cultivation was similar independently of the type of carbon source or the inoculation volume (Figure 3 A and C). The growing rate was maximal when 10% (v/v) sucrose was used. In this case, the maximal biomass was obtained after approximately 100 hours. The slowest growing rate was obtained when 5% (v/v) glucose was used (the maximal biomass was obtained after approximately 190 hours). As expected, similar results were obtained for the GFP signal measurement (Figure 3B).

These results show that the BioLector instrument can be used to optimize culture conditions. Continuous shaking during measurements ensures continuous mass transfer and homogenization of the suspended cells. The light scatter measurements are suitable for heterogeneous and large cell aggregates of plant suspension cultures. Moreover, the system allows for high-throughput screening of up to 48 batches in one microbioreactor system. In summary, the system enables the evaluation and discrimination of different cultures and therefore improves screening conditions, process development and scale-up procedures.
Monitoring Cell Growth
Cell size is a key parameter used to gain insights into various cellular mechanisms (e.g., cell cycle, osmotic regulation, cell death, pathogenesis, phagocytosis, species diversity, etc.). The Multisizer 4e uses the Coulter method to detect particles from 200 nm to 1,600 μm regardless of the particle’s nature or optical properties. Particles suspended in a 0.9% electrolyte solution are drawn through a small cylindrical aperture. Two submerged electrodes located on each side of the aperture create an electric current. As each particle passes through, it displaces its own volume of conducting liquid momentarily increasing the impedance of the aperture. This produces a voltage pulse that is proportional to the volume of the particle. The number of electrical pulses indicates the number of particles, while the amplitude of the electrical pulse depends on the particle’s volume.4 This allows researchers to accurately determine the volume, number, and cell concentration in a sample and detect real-time size changes. The latter is especially useful to detect cell aggregates that normally occur in plant cell cultures.5 In the following example, the Multisizer 4e instrument was used to evaluate the range of cell sizes in a plant cell culture.
Methods
A sample of the plant cell culture was pre-diluted 1:200 with Isoton 2 in a 400 mL beaker. From this dilution, 500 μL was used for each measurement. The aperture used was 1000 μm and each measurement lasted 120 seconds. The complete list of settings is shown in Table 1. The generated data were processed using Digital Pulse Processing (DPP) technology which enables the acquisition, storage and display of each individual pulse. This means that individual areas of the pulse spectrum can be evaluated separately at a later date.
Results and Discussion
The DPP data of the experiment shown in Figure 4 indicates that the size of the plant cells in this culture ranged between 30 and 320 μm.
From this raw data it is possible to select a range of interest (dotted lines in figure 4) and create different frequency histograms (i.e. the number of cells for each volume). For example, figure 5 shows the resulting histogram if a range between 40 and 140 μm is selected.
This data has an extensive range of applications. For example, the evaluation of samples at different time points can be used to study how cell size changes over time and can help to characterize the formation of cell aggregates.5 Additionally, the comparison of cell size obtained using different environmental conditions can help to optimize cell culture conditions. The DPP technology provides ultra-high resolution and accuracy (i.e. detection of 1 particle in 1 mL of a sample), which is unattainable using other technologies.
Conclusion
The BioLector microbioreactor and the Multisizer 4e instrument are bench-top devices with an intuitive user interface that can be integrated into any laboratory to optimize and monitor cell plant cultures.
References
- Loyola-Vargas VM, Vázquez-Flota F. An Introduction to Plant Cell Culture. In: Loyola-Vargas VM, Vázquez-Flota F editors. Plant Cell Culture Protocols. Methods in Molecular Biology, vol 318. Humana Press. 2006. https://link.springer.com/protocol/10.1385/1-59259-959-1:003#citeas
- Wani MC, Taylor HL, Wall ME, et al. Plant antitumor agents. VI. Isolation and structure of taxol, a novel antileukemic and antitumor agent from Taxus brevifolia. J Am Chem Soc 1971:93(9):2325-2327. doi: 10.1021/ja00738a045
- Georgiev MI, Weber J, Maciuk A. Bioprocessing of plant cell cultures for mass production of targeted compounds. Appl Microbiol Biotechnol 2009;83:809-823. https://link.springer.com/article/10.1007/s00253-009-2049-x#citeas
- Rhyner M, Prestigiacomo G, Kumar K, Lee L. Cellular Analysis using the Coulter Principle. Measurement with Great Accuracy and Speed. Available from: https://www.mybeckman.uk/resources/reading-material/application-notes/cellular-analysis-using-the-coulter-principle
- Kolewe ME, Henson MA, Roberts SC. Characterization of aggregate size in Taxus suspension cell culture. Plant Cell Rep 2010;29(5):485-494. doi: 10.1007/s00299-010-0837-5